The Soul of Formula E
DECEMBER 7, 2021
Written by Dr Oliver Watkins
The Soul of a Series?
When I was a much younger man, the F1 team I was working for decided that it would be a good idea to have a regular quiz on the technical regulations of F1. The idea behind this was that if the staff were well informed on the regs they would be able to innovate their way to imaginative car upgrades which respected the regulations as written but achieved something quite different. In the event, however, all the questions were along the lines of “what is the upper extent of the legality box for the front wing endplates, relative to the reference plane?”. Knowing this, I thought, was unlikely to stimulate the mind of the reader to come up with another F-duct, E-Shift gearbox or J-Damper, all of which were pioneered during my time. I therefore gave up on the quiz in pretty short order.
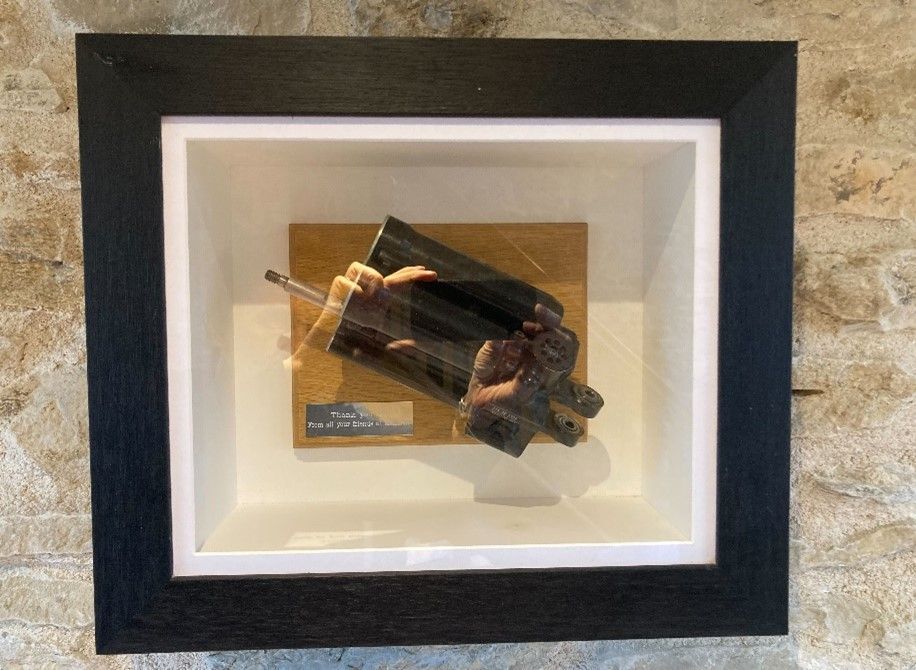
My leaving present, which I certainly didn’t dream up as a result of knowing how big the DRS slot gap was allowed to be. This component ran on both cars for several years. What it does will have to remain a mystery.
The point of this misty-eyed reminiscence is that technical regulations tend to fall in to two categories:
The rather boring ones, which define where your physical components can live.
The interesting ones which really have an impact on the “soul” of your car.
From my highly biased viewpoint, a neat proxy for this is that the interesting components are the ones which come to the attention of your simulation development and modelling team. For instance, the position of the front wing endplates; well, that’s just going to have a modest impact on your aeromap. Adding the F-duct, however, adds an entirely new driver control that needs to be understood and tamed.
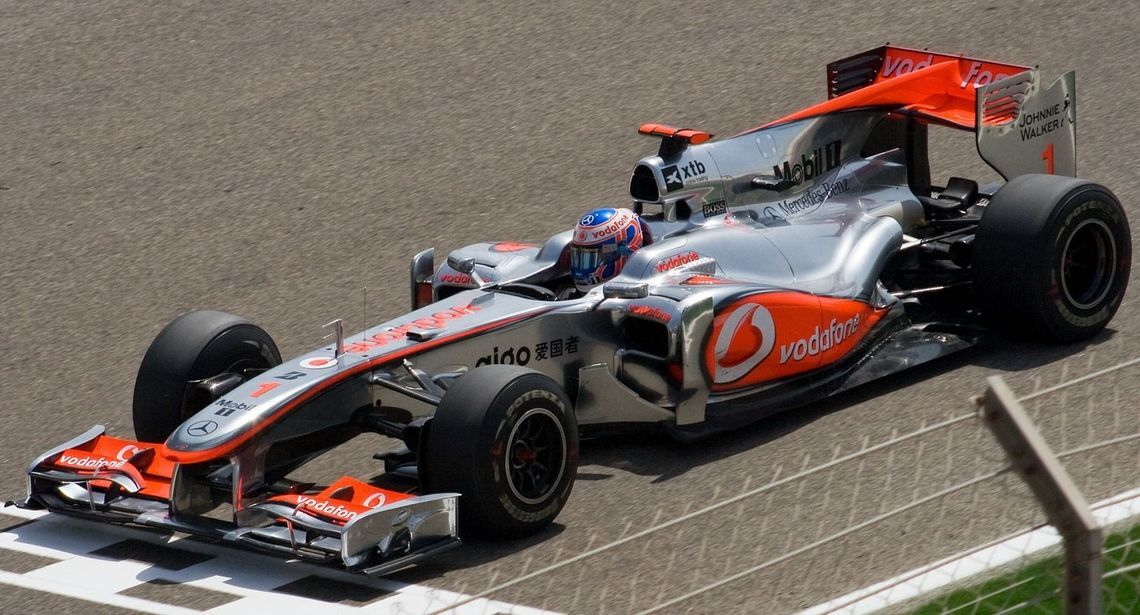
The F-duct; a brilliant innovation from a brilliant mind.
In order to capture the “soul” of each series, therefore, we have decided to write a set of blog posts describing what makes each series interesting from a simulation point of view, and how these simulation challenges are best addressed. We’re going to start this set of articles with a look at Formula E, a formula very close to Canopy’s heart, where we supply a significant majority of the grid with simulation tools.
It’s All About Energy
In Formula E, or FE, for short, it’s the “E” that does all the work. Success or failure is heavily dependent on electrical energy, and what you do with it. The battery of the 2nd Generation cars can deliver about 54kWh (or 194.4 MJ) of energy. While this seems like a lot, it’s nowhere near enough to go flat out for the whole race, and that’s the single biggest challenge the teams have to face.
Teams who are to succeed in Formula E must eke every bit of value of out every Joule of energy they have at their disposal. The big-ticket items here are the deployment and regeneration efficiency of the electric motor (soon to be two electric motors), and even more critically, where the drivers choose to use their limited energy.
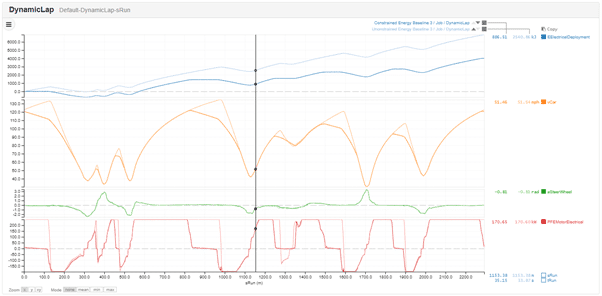
There’s world of difference between having enough energy to go flat out and having to ration your energy carefully.
Looking at the picture above, we can see that there are 4 distinct phases to each corner of the track:
Mid-corner; go as fast as grip will allow.
Corner exit; accelerate as hard as possible.
Mid-straight; lift off the accelerator pedal and begin coasting.
Near corner; pull the regen paddle and start actively harvesting energy from the rear axle.
In the bad old days, the only way to determine the optimal energy deployment strategy was first to take a guess at the best strategy (and not all teams converged on the 4-phase strategy shown above). Having guessed what your strategy should be, some poor soul would then have to search long-hand for the lift off points and regen points which gave the fastest lap time while respecting the energy constraints. In the example above there are 14 such points, so finding the optimal combination rapidly becomes a formidable combinatorial problem.
To the great relief of many simulation engineers Canopy now solves this problem in one hit; the simulations shown above each took about 5 minutes (although we parallelize across machines in the cloud, so running both simulations also took 5 minutes). We don’t feed the simulation any preconceived ideas about what strategy to follow; it just does whatever is fastest. The problem left to the teams then is to distil this knowledge into instructions for the driver, and to persuade the driver to follow them. And follow them he must; deviating from the optimal strategy gets very costly, very quickly. This applies both in terms of the case where the driver decides to invent his own lift-off points, and also when they progressively “borrow” energy from the future. At some point this has to be paid back, and doing so will be damaging in terms of lap time.
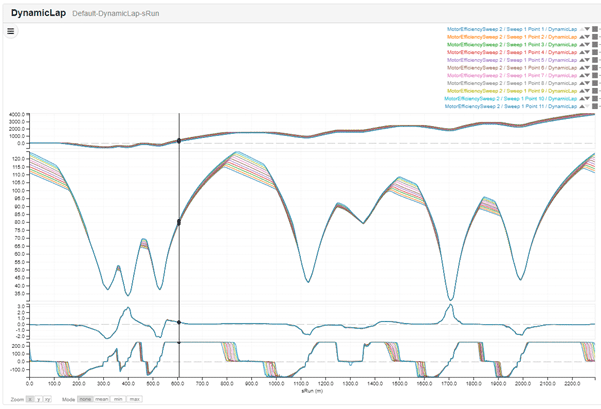
Sweeping from 100% motor efficiency to 90% efficiency shows the debilitating effect of reduced motor efficiency in an energy limited situation. Some performance is lost under acceleration, but the losses while coasting are crippling.
With the energy deployment problem now solved, we can discover the sensitivities to (for instance) motor efficiency, as shown in the sweep above. The penalty for a 10% loss in motor efficiency is a troubling 2.2 seconds on this circuit; as much again as the cost of going from unlimited energy to a usage pattern that allows teams to finish the race. By contrast, if energy is unlimited the same 10% change in efficiency will cost “only” 0.75s. This difference is so large because dissipating more energy as heat kicks off a vicious cycle which “gears up” the effect of the losses:
Dissipating energy as heat under acceleration kicks off a vicious cycle of losses which cause much large losses than when there is plenty of energy to go around.
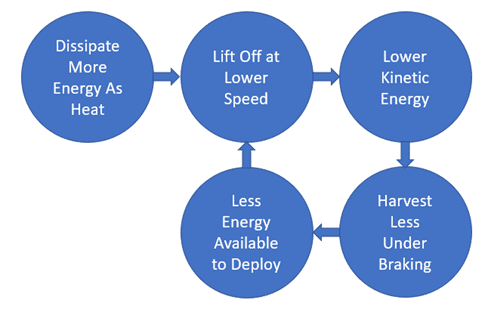
The same principles apply throughout the car; anywhere that energy is unnecessarily lost will cause outsize losses in lap time. If, therefore, your driver is unwisely sliding the tyres more than is necessary on corner exit, he is dissipating energy unnecessarily and will pay a lot more for it than his counterpart in F1.
Surely, I hear you cry, the charging and discharging efficiency of the battery is of critical importance? Surprisingly the answer to this is “yes and no”. The FIA have mandated that the round-trip efficiency of the battery cannot exceed 75%, so if you harvest 1MJ of energy under braking, only 750kJ of this can be added back on to your allowance for the rest of the race. Therefore, if your battery is so inefficient that you risk running out of electrons in the battery, you’re in big trouble and battery efficiency is a big deal. On the other hand, if you are doing better than 75%, then the true efficiency is large irrelevant to you. The kicker here is that the batteries are supplied by a single supplier with an excellent round trip efficiency, so it’s not a big concern for the teams. There is a big exception to this: when it gets hot the challenges faced by the teams multiply rapidly; we cover this in more detail in the next section.
It’s Getting Hot In Here
As well as being the title of a truly execrable song by “Nelly” from 2002, it’s also likely to bring Formula E engineers out in an ironic cold sweat. Since the advent of the Gen2 Formula E car, thermal management has become much less of an issue, but this is not to say that it’s no longer a concern.
The battery of a Formula E car has three significant derates (conditions where the performance of the battery is restricted), all based on not doing things to the battery that are likely to be damaging:
A high State-Of-Charge derate; don’t harvest energy when the battery is already full (very sensible).
A low State-Of-Charge derate; don’t deploy energy when the battery is nearly empty (also sensible).
A thermal derate; don’t harvest or deploy energy when the battery is too hot (extremely sensible; getting the battery really hot could cause a thermal runaway and a very large fire).
The first two of these are fairly easy to manage; ration your energy sensibly over the laps you have to complete, and all will be well. The 3rd , however, is much more problematic; the temperature of the battery at the end of the race will be somewhat dependent on how you drive at the start of the race. You can therefore easily bake in a catastrophic loss of performance at the end of the race, without realising. Anyone who has started a running race far too fast will know this feeling; there’s a long way to go and there is no recovering from the mistake you’ve already made. Even worse than this, the efficiency of the battery tends to drop as the state-of-charge decreases, meaning that just as the battery is getting really hot, and you desperately need to dump less in heat into it, keeping on racing will do precisely the opposite, and potentially in quite a big way.
This is the sort of situation that simulations like ours, which can trade deployment on lap 1 against temperature on lap 30, were made for. The example below is a bit boring, because despite getting hot, the battery doesn’t start to derate. What you can’t see is that the simulation adapts to the fact that the big constraint is now avoiding hitting the derate temperature, rather than the energy constraint. This is rather frustrating really; finishing a race with some electrons left in the battery just doesn’t feel right, especially given what we’ve said earlier in the article!
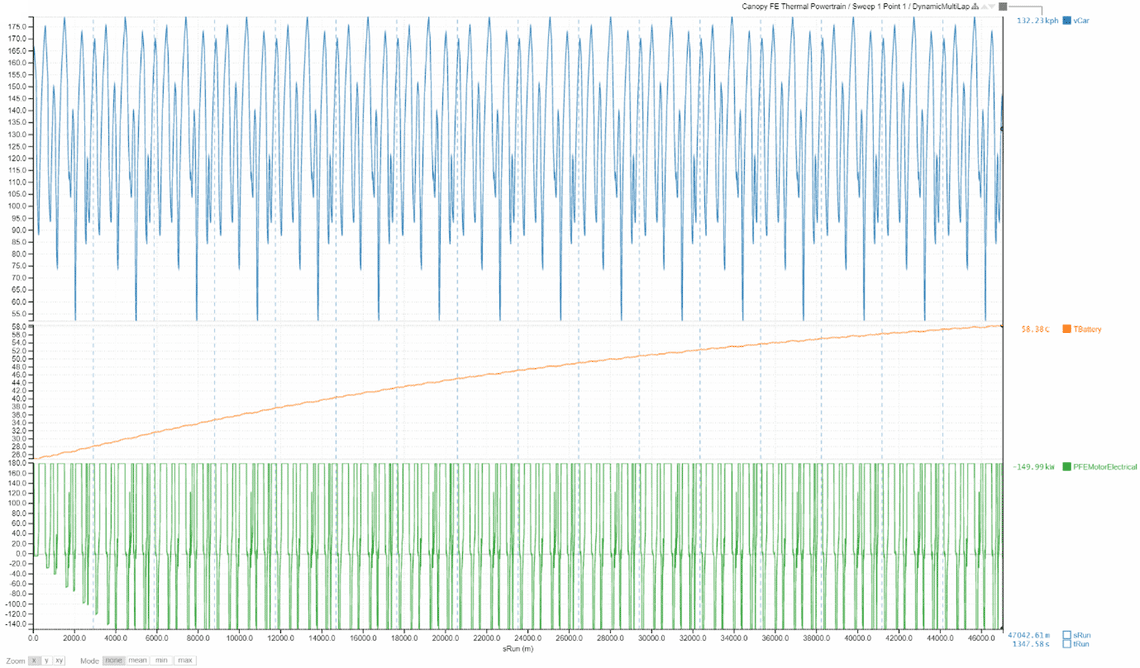
Not the most thrilling example, but simulating an entire stint in one go allows us to trade performance on lap 1 against performance right at the end of the race, 45 minutes later.
Attack Mode
In the absence of pitstops, FE has found some other interesting means of spicing up the show. Attack Mode allows the cars to use a maximum of 300kW, rather than 250kW, for a 4 minute period. This is enabled by running off line through a trigger zone. If you wish to do this in the best way possible you probably want to answer three questions:
How much time does it cost to go through the trigger zone?
How much time do I gain over the next 4 minutes?
How much electrical energy should I “steal” from other laps of the race to use during Attack mode?
Once again, we’re in a situation where we’re trading off performance on lap 1 against performance many laps later, so we need an approach of “optimize everything at once”. Fortunately, Dynamic Multi Lap can come to the rescue again. For this example we’ve moved tracks to Marrakesh, and demanded that the car go wide at the apex of turn 2 to trigger Attack Mode for 4 minutes.
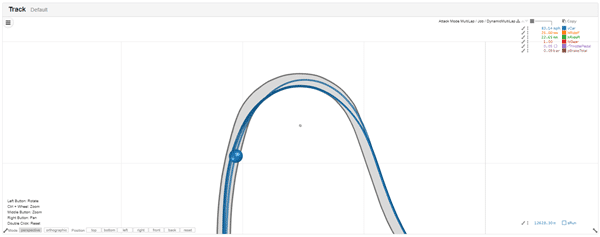
Go wide at the entry to turn 2 to get 4 minutes of 300kW maximum power.
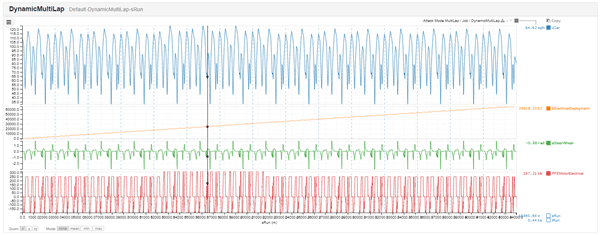
15 laps of Marrakesh with a dose of attack mode starting on lap 5.
The simulation has to choose what to do with 79MJ of energy in this simulation. The key features of the results are:
Laps without attack mode enabled: 5.27MJ per lap, with a lap time of 79.25s.
Laps with attack mode enabled: 5.5MJ per lap with a lap time of 78.15s.
Lap time without using attack mode at all: 79.15s.
This shows that when using attack mode the car should use more energy, and to do so yields a lap time a full 1.1s faster than during conventional running; far more than the 0.2s cost of going off line to trigger attack mode. It also shows that borrowing energy from the laps when we don’t have attack mode will cost 0.1s per lap, which is probably manageable.
Conclusions
So there, we think, we have it; the soul of Formula E from our wildly biased simulation perspective. Next year will bring the 3rd generation cars, about which we have blogged before. We believe these will change the nature of the racing and the control of the cars to a limited degree, but won’t fundamentally alter the soul of the formula. However, if the nature of the spectacle changes, we’ll revisit this article to understand and explain why it has changed.
Next in the series we’ll be tackling the daddy of them all: F1. In the meantime you may enjoy reading our blog on Battery Thermal Management in Formula E.